Oxygen Transport and hypoxia
Podcast episode
Take home messages
- Oxygen is rather important
- All hypoxia is not equal
We like oxygen
Generally speaking, the old O2 is a force for good in the world of anaesthesia.
So we tend to worry when our patient isn't quite getting enough of the good stuff.
How is oxygen content in arterial blood estimated?
The 'amount bound to haemoglobin' is calculated by:
- Hb concentration x % saturations x Hüfner's constant
- Where Hüfner's constant is between 1.34 and 1.39 ml O2/g Hb
- (Hüfner's constant is just how much oxygen each unit of haemoglobin can carry)
The 'amount dissolved in plasma' is:
- The partial pressure of oxygen x 0.023ml/100ml
- The relevant gas law to mention here is Henry's law - where the amount of dissolved gas in solution is directly proportional to the partial pressure of the gas above the liquid
So the overall equation looks like this
Don't get caught out by the units in this equation - the haemoglobin concentration is in 'old units' - so around 10-15 g per 100ml
A bit about haemoglobin
If you take some of the wondrous chlorophyll that paints the earth green, swap out the magnesium for iron and then lop off a couple of hydrocarbon tails, you get the equally ubiquitous haemoglobin.

Describe the structure of haemoglobin
- Four polypeptide chains
- Two alpha and two beta chains
- Bound by hydrogen bonds
- Four haem moieties or porphyrin rings each with an iron atom at the centre
- Iron in its ferrous state
So how does it work?
The oxygen molecule lands in the blood and bumps into a haemoglobin molecule, at which point it reversibly binds to the iron atom in its ferrous state.
Four oxygen molecules (eight atoms) can bind to each haemoglobin molecule, and they do this in a manner called cooperative binding via the process of allosteric modulation.
This means that as one oxygen molecule binds to one haem moeity, it induces a change in the quaternary structure of the haemoglobin molecule, by bringing the beta chains closer together (called the relaxed state).
This relaxed state has a higher affinity for oxygen, and so the next three molecules can all bind more easily.
Once the haemoglobin molecule gets to the tissues, and the oxygen dissociates, the haemoglobin returns to the taut conformation, with a reduced affinity for oxygen, to prevent those same molecules from snapping back on board, and facilitating oxygen delivery to the hungry tissue cells.
This is why the oxyhaemoglobin dissociation curve has a sigmoid shape - there is initially a low affinity for the first oxygen molecule to bind, requiring a higher partial pressure to increase percentage saturations, but as soon as one binds, the next three bind in quick succession with very little increase in partial pressure - hence the sudden steepness in the curve.
Time for a classic FRCA OSCE/SOE question:
Please draw and explain the oxyhaemoglobin dissociation curve
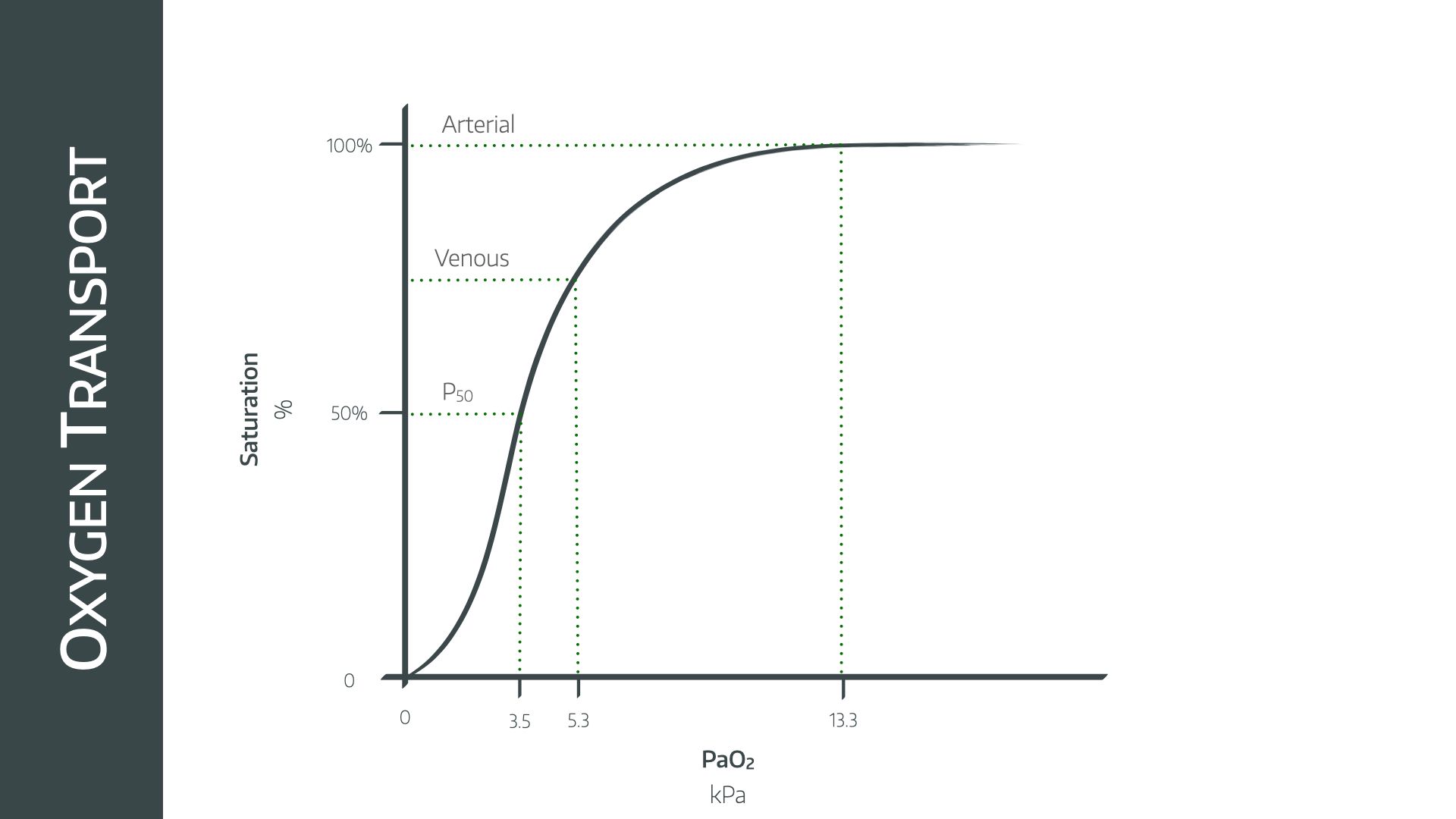
This is a sigmoid graph as a result of cooperative binding demonstrated by the four oxygen molecules and haemoglobin.
The key points on the graph
The arterial point
- 97-100% saturations at a partial pressure of 13.3kPa
The venous point
- 75% saturations and 5.3kPa
The P50
- The P50 is the partial pressure of oxygen at which haemoglobin is 50% saturated, usually at 3.5kPa
- It is used as the reference point to determine the degree of left or right shift
You might be asked to add a curve to represent myoglobin, which can only carry one molecule of O2. This would be a hyperbolic graph (almost straight up and then across) drawn to the left of the sigmoid haemoglobin graph.
What factors affect the position of this curve?
Factors that increase the affinity of haemoglobin for oxygen - favouring oxygen uptake - will shift this curve to the left.
- Low CO2
- Alkalosis
- Hypothermia
- Foetal haemoglobin - P50 of foetal haemoglobin is 2.5
- Reduced 2,3-DPG
- Carbon monoxide
- Methaemoglobinaemia
Factors that decrease affinity - and therefore promote unloading of oxygen to the tissues - will shift this curve to the right.
- Right shift (Lower O2 affinity, favouring unloading of oxygen)
- High CO2 (Bohr shift)
- Acidosis
- Hyperthermia
- Increased 2,3-DPG
- Pregnancy
- Altitude - Partly via increased 2,3-DPG production
- Sickled haemoglobin
Left and right shift is technically measured using the P50.
How is 2,3-DPG produced and what does it do?
- Stands for 2,3-Diphosphoglycerate, an organic phosphate molecule produced by red blood cells
- 2,3-DPG causes a right shift in the oxyhaemoglobin dissociation curve, promoting unloading of oxygen to the tissues
- It neatly allows the red blood cell to 'choose' between energy production and oxygen unloading, depending on the needs of the tissue it supplies
- The red blood cell can either continue the glycolysis pathway, producing 3-phosoglycerate, or it can produce 2,3-DPG and prioritise oxygen unloading instead
Packed red cells have little-to-no 2,3-DPG, and so their ability to offload oxygen is fairly rubbish until they've warmed up and got hold of some sugar in the recipient's bloodstream.
2,3-DPG production requires phosphate, hence the importance of correcting hypophosphataemia in critically unwell patients.
The O2 Cascade
If you want molecules to flow, you need a concentration gradient - or for a gas you need a partial pressure gradient - down which they can slide.
In the case of oxygen, the top of our slide is the atmospheric air outside the nose and mouth, and the bottom is the patient's mitochondria.
The oxygen cascade is the name given to the stepwise reduction in partial pressure of oxygen between the atmosphere and the mitochondrion via each step of the cardiorespiratory system.
And you need to be able to draw it.
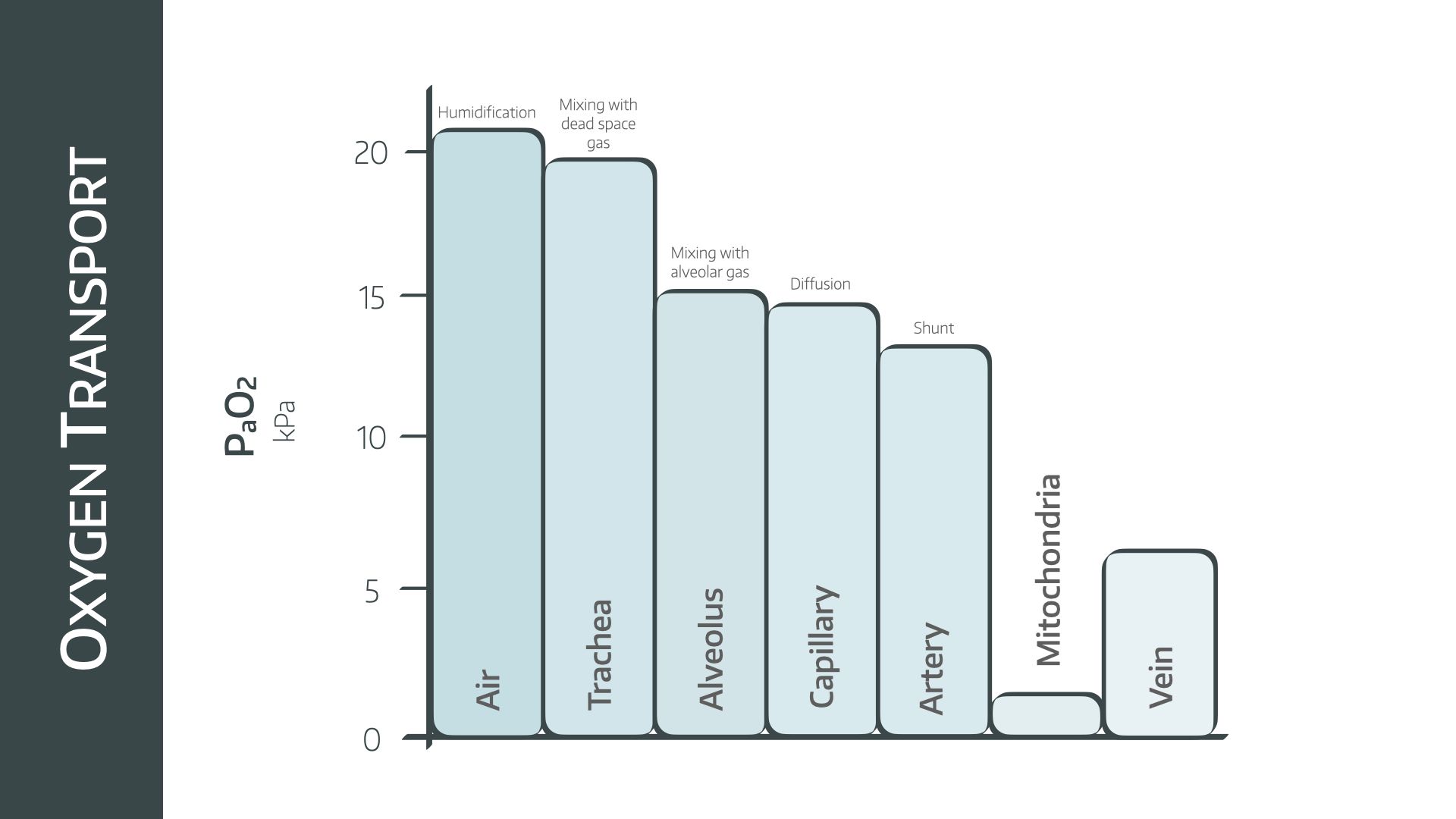
Starting with room air
- PO2 = Patm x FiO2 = 101kPa x 21% = 21kPa
As the air enters the trachea it is humidified and warmed, reducing the partial pressure of oxygen due to the saturated vapour pressure of water
- PO2 = FiO2 x (Patm - PH2O) = 21% x (101 - 6) = 19.95 kPa in the trachea
The air then travels down from trachea to bronchioles to alveoli, where it mixes with CO2 from the alveoli. The alveolar partial pressure of oxygen is estimated by the alveolar gas equation
- PAO2 = 21%(101 - 6) - 5.3/0.8 = 13.3 kPa in the alveolus
There is a small drop in PaO2 due to diffusion barrier into the pulmonary capillaries.
The capillaries then mix with shunted blood to form arterial blood.
This classically reduces the PaO2 by approximately 0.5-1.0 kPa.
There is then a steady consumption of oxygen as blood passes through arteries, arterioles and capillaries until the oxygen finally reaches the mitochondria with a final partial pressure of 1.5 kPa.
What is the Pasteur point?
This is the minimum partial pressure of oxygen, inside the mitochondrion, at which oxidative phosphorylation is still able to occur
- It is around 0.13 kPa or 1mmHg
We can therefore see just how crucially important each step in the cascade is for determining whether or not the body is able to perform oxidative metabolism, and leads us nicely onto our categories of hypoxia.
What are the types of hypoxia?
Hypoxia is defined as an insufficient oxygen supply to meet the demands of the tissues for normal cellular function, and as a result the tissues have to resort to lactate-pumping anaerobic metabolism, which can only continue for so long.
When considering hypoxia, it makes sense to think back to the the oxygen cascade - the bumpy partial pressure gradient down which the oxygen molecules slide to their mitochondrial destination.
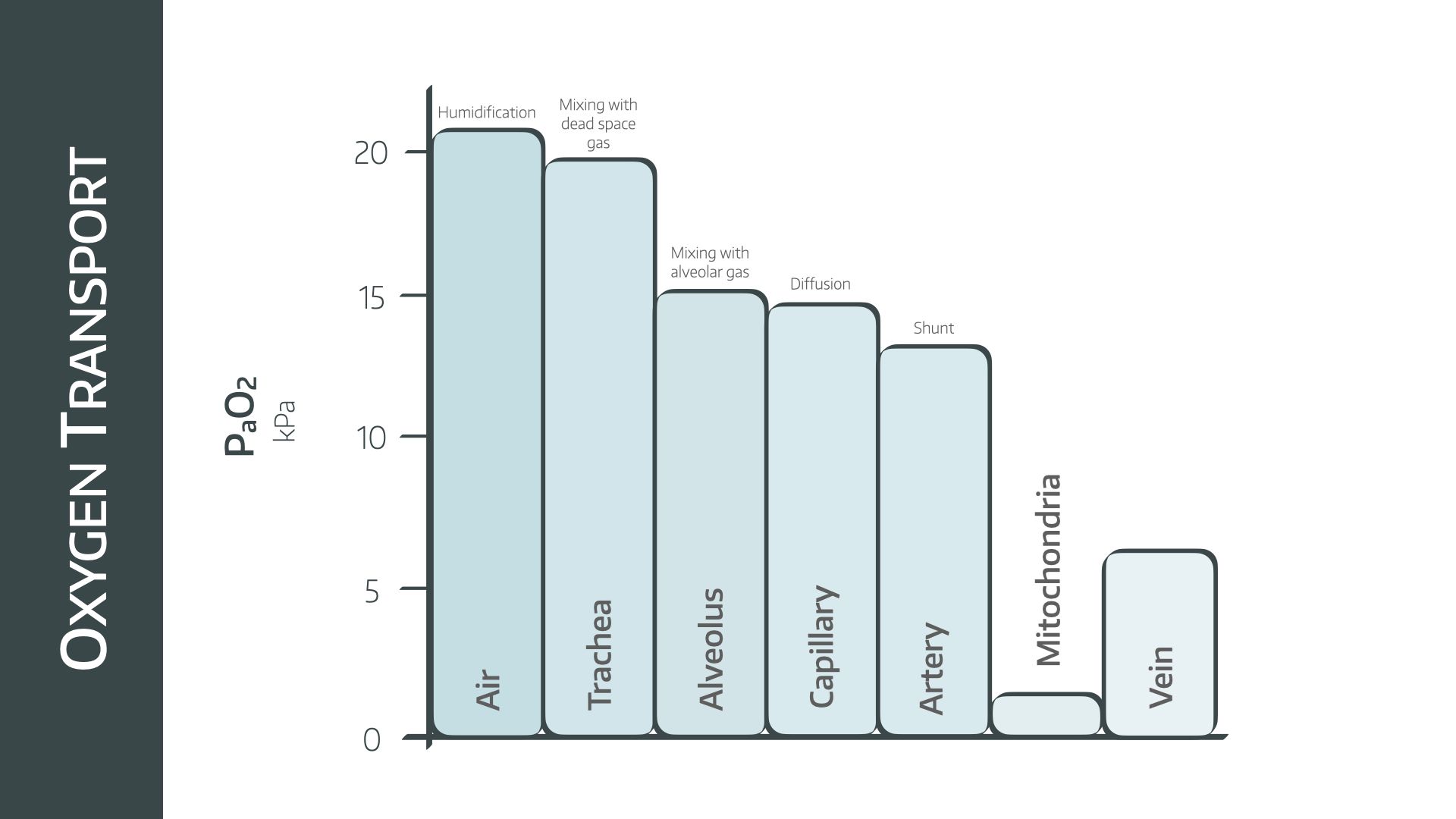
Now hypoxia can occur at any point along this cascade and it can be systemic or regional.
So here's the ultra-popular exam question that you almost certainly will get asked at some point in your exam career.
What are the types of hypoxia?
There are four categories of hypoxia - hypoxic, ischaemic, anaemic and histotoxic
Hypoxic
This is a pathological reduction in the partial pressure of oxygen in the blood, leading to hypoxaemia and low haemoglobin saturations.
- Hypoventilation
- Reduced FiO2
- VQ mismatching
- Gas transfer impairment (see our article on A-a gradient)
Ischaemic
Also known as stagnant hypoxia - the blood has enough oxygen content, but the blood isn't being delivered to the tissues effectively.
This can be a regional issue if a certain part of the body is affected, or systemic if there is generalised cardiac output or delivery failure.
Regional
- Limb ischaemia due to peripheral vascular disease or compartment syndrome
- Tourniquet
Systemic
- Microcirculatory failure due to sepsis
- Cardiac failure causing stagnation of blood
- Any organ failure syndrome involving shock
Anaemic
Here there is a reduction in the blood’s oxygen carrying capacity as a result of reduced functional or total amounts of haemoglobin.
The partial pressure of oxygen dissolved in the blood may well be normal, but there simply isn't enough functional haemoglobin to carry sufficient amounts to the tissues.
This means the available haemoglobin is usually fully saturated, giving falsely reassuring saturations readings on the monitor.
- Severe chronic anaemia (absolute Hb reduction)
- Carbon monoxide poisoning (functional Hb reduction)
Some sources will put CO poisoning in the histotoxic section. It can probably be argued both ways, but in my head it makes more sense to put it here because the Hb can’t bind to the oxygen, rather than the tissues not being able to absorb it from the blood.
Histotoxic
There's enough oxygen in the blood, and it's being delivered to the tissue, but for whatever reason the mitochondria cannot access or use the available oxygen, and the cell has to resort to anaerobic respiration.
Patients frequently have high venous oxygen saturations, as they cannot remove the oxygen from the haemoglobin and so it simply travels around the cardiovascular system.
- Cyanide (uncouples oxidative phosphorylation)
- Severe sepsis (microcirculatory failure)
- Malignant hyperthermia
Technically malignant hyperthermia demonstrates three of the types:
- histotoxic hypoxia as a result of mitochondrial failure
- ischaemic hypoxia due to localised compartment syndrome induced by muscle swelling
- hypoxic hypoxia as a result of excessive oxygen demand
The importance of understanding these different types lies in their management.
Hypoxic hypoxia requires more oxygen, but just increasing the FiO2 in any of the other types isn't going to help.
- Ischaemic hypoxia needs better perfusion or cardiac output
- Anaemic hypoxia needs more functional haemoglobin
- Histotoxic hypoxia needs the underlying cause treating
What factors increase and decrease VO2?
Increase
- Pain
- Exercise
- Shivering
- Sepsis
- Trauma
- Hyperventilation
Decrease
- Hypothermia
- Sedation
- Analgesia
- Mechanical ventilation
- Severe shock
Syllabus
- PB_BK_57 Gaseous exchange: O2 and CO2 transport, hypoxia and hyper- and hypocapnia, hyper- and hypobaric pressures
- PB_BK_58 Function of haemoglobin in oxygen carriage and acid-base equilibrium
Useful Tweets
Clinical targeting of the cerebral oxygen cascade to improve brain oxygenation in patients with hypoxic–ischaemic brain injury after cardiac arrest
— Critical Care Reviews (@CritCareReviews) July 28, 2023
CCR Journal Watchhttps://t.co/Sp06oA6IDG
Get the latest critical care literature every weekend https://t.co/nitMzacLrj pic.twitter.com/j9XvN9PBnf
References and Further Reading


Primary FRCA Toolkit
Members receive 60% discount off the FRCA Primary Toolkit. If you have previously purchased a toolkit at full price, please email anaestheasier@gmail.com for a retrospective discount.
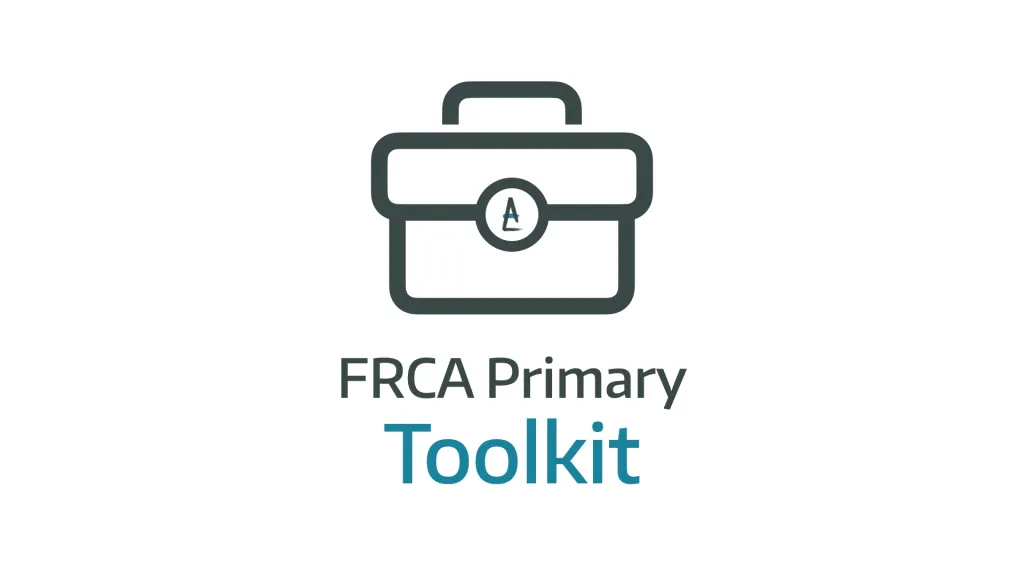
Discount is applied as 6 months free membership - please don't hesitate to email Anaestheasier@gmail.com if you have any questions!
Just a quick reminder that all information posted on Anaestheasier.com is for educational purposes only, and it does not constitute medical or clinical advice.