CO2 Handling
Take home messages
- CO2 is a waste product with profound cardiorespiratory effects
- It's also a useful measuring tool
- Don't forget the effects on oxygen via the alveolar gas equation
A useful waste product
Carbon dioxide is a waste product of aerobic metabolism, and we don't really want it to hang around in the body because it can have a variety of sub-optimal effects.
Where does CO2 come from?
- CO2 is a metabolic waste product produced in the citric acid cycle in the mitochondrion in metabolically active cells
- It is produced at around 200 ml/minute at rest*
- There are approximately 120 litres of CO2 in the body at any given point, or 1.8 litres per kilo of body weight
*The two factors that affect the rate of CO2 production are:
- The rate of aerobic metabolism
- The respiratory quotient (which depends on what fuel is being metabolised)
For a respiratory quotient of 0.8, and 250ml/minute oxygen consumption, this gives us 200ml/minute of CO2 production
Effects of raised PaCO2
Respiratory
- Respiratory acidosis
- Right shift in oxyhaemoglobin dissociation curve
- Bronchodilation
- Pulmonary artery vasoconstriction
Cardiovascular - largely due to sympathetic stimulation
- Vasodilatation
- Hypertension
- Tachycardia
- Catecholamine release
- Increased cardiac output
- Prolonged QT interval
Neurological
- Narcosis
- Vasodilatation and raised intracranial pressure
- Depressed airway reflexes
Renal
- Increased bicarbonate
- Increased renal vascular resistance
- Reduced renal function
So yes it's a waste product, but that doesn't mean it has to go to waste - we can still use it
The vast majority of CO2 is excreted from the body via the lungs, which makes it very useful as a measuring tool for several of our favourite parameters in anaesthesia:
- Confirmation of airway patency
- Cardiac output
- Metabolic status
From Cell to Air
Given CO2 has rather profound cardiovascular, respiratory and haematological effects, we need a way to safely package and transport it from the cell to the lungs.
How is CO2 transported around the body?
There are three main transport mechanisms that you need to know about:
- Transport as bicarbonate
- Transport as carbamino compounds
- Dissolved in the plasma
The vast majority is transported as bicarbonate, and the proportions vary depending on whether you're looking at arterial or venous blood.
- Arterial - 90% bicarbonate, 5% carbamino compounds, 5% plasma
- Venous - 60% bicarbonate, 30% carbamino compounds, 10% plasma
Transport as bicarbonate
The reversible equation you need to know looks like this:
CO2 + H2O ⇌ H2CO3 ⇌ H+ + HCO3-
Now this reaction will occur spontaneously, but the enzyme carbonic anhydrase is used to speed things up.
Carbonic anhydrase is found within the red blood cells, and fortunately, CO2 can quite happily diffuse across the cell membrane to get to it. Once inside the cell, the resulting positive and negative ions become trapped inside the cell, which is helpful for prevent wild swings in plasma pH.
However there's an issue - the build up of Hydrogen (H+) and bicarbonate (HCO3-) ions in the cell will cause problems:
- The osmotic impact of increasing ion concentration will make the cell swell with water and burst
- The increasing concentration of bicarbonate will inhibit the forward reaction and slow down the conversion of CO2 to bicarbonate, so we need to try and get rid of it.
To achieve this, bicarbonate is swapped for equally negative chloride ions (to maintain the electronegative balance inside the cell) in a process known as the chloride shift.
What about the build up of hydrogen ions you ask? - this gets mopped up by the fabulous buffer that is haemoglobin.
Here's a video
Transport as carbamino compounds
CO2 will quite happily bind to certain chemical groups, and has a particular affinity for amine groups.
R−NH2 + CO2 ⇌ RNH − CO2 + H+
Most proteins will have a terminal amine group or two hanging around somewhere, and so can pick up a few molecules of CO2, but the the main player in this game is reduced or deoxygenated haemoglobin.
The reason for this is that reduced haemoglobin has a unique ability to bind and buffer those H+ ions released by the equation above, thanks to the large number of histidine residues (38 per haemoglobin tetramer).
The hydrogen ions bind to the imidazole groups of these histidine residues, and this makes reduced haemoglobin the main protein buffer of hydrogen ions.
Deoxygenated haemoglobin has a pKa of 8.2, while oxygenated haemoglobin has a pKa of 6.6.
Here's a quick reminder about pKa
Transport in plasma
CO2 will quite happily dissolve in water, of which there is rather a lot in plasma.
If you've ever wondered why pulmonary oedema causes a predominantly type I respiratory failure but doesn't seem to impact CO2 clearance so much - this is why - CO2 will merrily dissolve across the alveolar membrane and through the alveolar fluid, but oxygen isn't so keen.
Henry's law states that the amount of gas dissolved in a liquid will be proportional to the partial of pressure of the gas above (or around) the liquid.
Solubility varies with temperature, with increasing temperature causing a reduction in solubility.
At 37°C, each 100ml of plasma contains around 0.5ml/kPa of carbon dioxide.
Can you draw the CO2 Dissociation Curve?
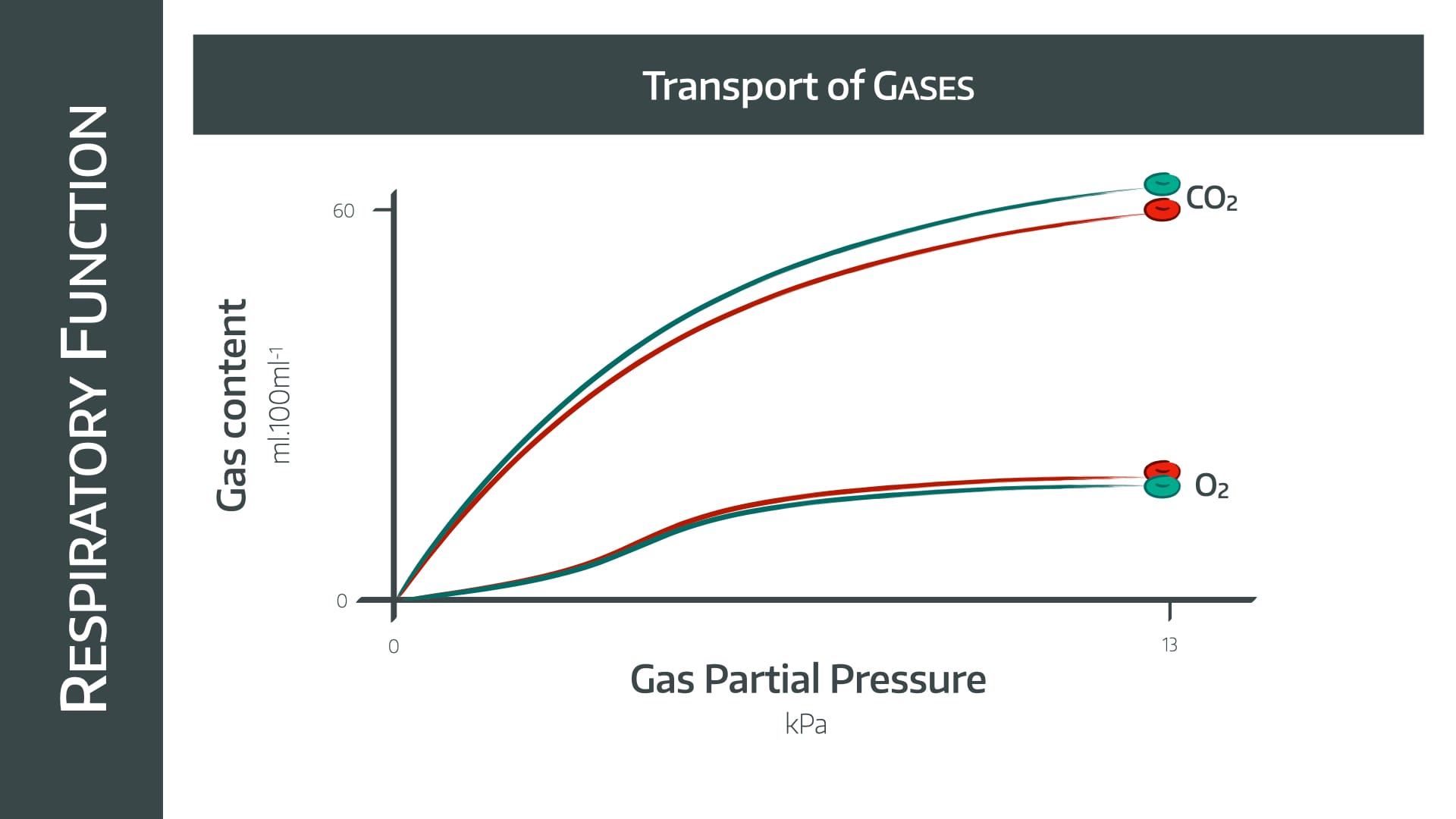
The difference seen between the two gases here is due to the difference in their solubilities.
CO2 is massively soluble, so if you increase the partial pressure of gas, then more and more will merrily dissolve away in the plasma (Henry's law)
Oxygen, however, is much less soluble, and relies on haemoglobin carriage to a much greater extent.
Therefore once the haemoglobin is fully saturated at around 11 kPa, any further increase in partial pressure of oxygen doesn't hugely increase oxygen content.
Tell me about the Haldane effect
The Haldane Effect describes the change in haemoglobin's affinity for CO2 with changes in partial pressure of oxygen (kind of the reverse of the Bohr effect).
Deoxygenated haemoglobin is three times more comfortable carrying CO2 than oxygenated haemoglobin, meaning:
- In areas with lots of oxygen, the affinity reduces and CO2 gets offloaded
- In areas with less oxygen, the affinity increases, and haemoglobin mops up CO2
Makes sense.
This offloading process is so effective that even though the volume of CO2 carried in the form of carbamino compounds is only around 20% of the total volume in the blood, it accounts for over 30% of the CO2 that actually gets exhaled.
What happens in the lungs?
Let's start with some assumptions that they'll expect from you in the exam:
- Normal arterial blood has around 5.3 kPa CO2
- Normal venous blood has around 6.1 kPa CO2
Ignore the red cells for a moment
This means 100ml arterial blood will contain around 2.5 ml of dissolved CO2 and the same volume of venous blood will have around 3ml CO2.
If we have an average adult cardiac output of 5 litres per minute, then we're looking at around 150 ml of dissolved carbon dioxide reaching the lungs each minute.
Around 25 ml of this will be exhaled.
Okay now think about the red cells
As the blood passes by the alveolus, the partial pressure of oxygen dramatically increases, and activates the Haldane effect, triggering unloading of CO2 molecules.
These then diffuse across into the alveolus and out through the respiratory tree.
CO2 is so good at diffusing through both water and air, that the PACO2 and PaCO2 are essentially identical, hence we make this assumption in the alveolar gas equation.
How does ventilation affect PACO2?
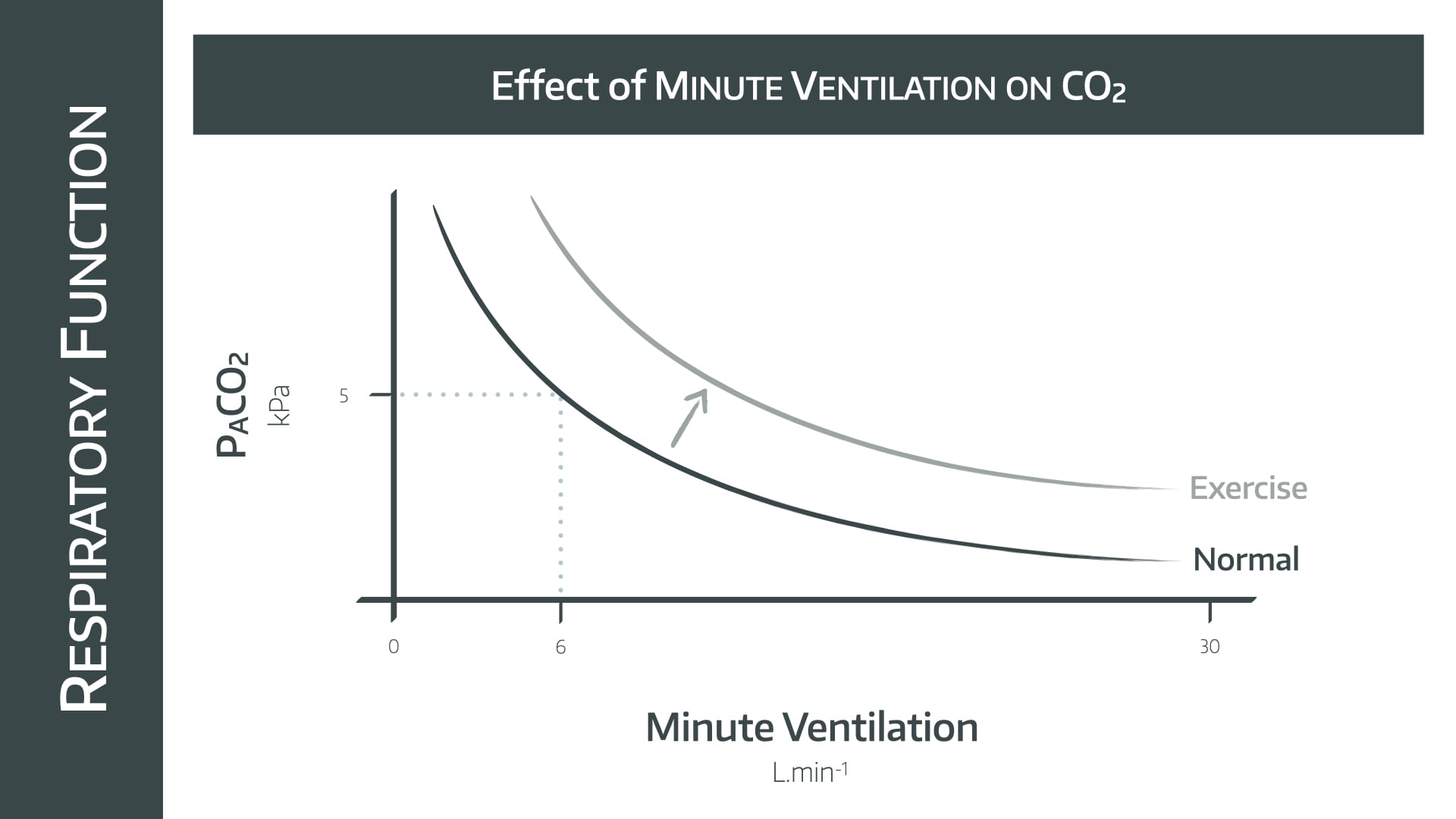
This is a graph you need to be able to draw in the exam.
- This is a rectangular hyperbolic graph
- As alveolar minute ventilation doubles, PACO2 halves
Use the reference point of 6 litres per minute ventilation maintaining a PACO2 of 5 kPa, and draw the graph out in either direction from that point.
During exercise the increase in aerobic metabolism is met by an increase in minute ventilation, shifting the graph upwards and to the right as displayed.
How does CO2 affect ventilation?
It's probably not news to you that CO2 drives the respiratory centre in the medulla, so an increase in PaCO2 will generally result in an increase in respiratory effort.
Assuming you've not reached the realms of narcosis quite yet.
But how does this happen?
CO2 dissolved in the plasma can very easily cross the blood brain barrier and dissolve in the CSF.
It is therefore able to trigger central and peripheral chemoreceptors, both of which input to the respiratory centre in the medulla.
- Peripheral chemoreceptors respond to PCO2 and pH via the carotid bodies (glossopharyngeal) and aortic bodies (vagus nerve)
- Central chemoreceptors respond to pH changes in the CSF and increase minute ventilation
Because the CSF doesn't contain much in the way of proteins or other buffers, very minor changes in CO2 concentration cause rapid and dramatic changes in pH, which trigger the sensitive receptors to increase respiratory effort.
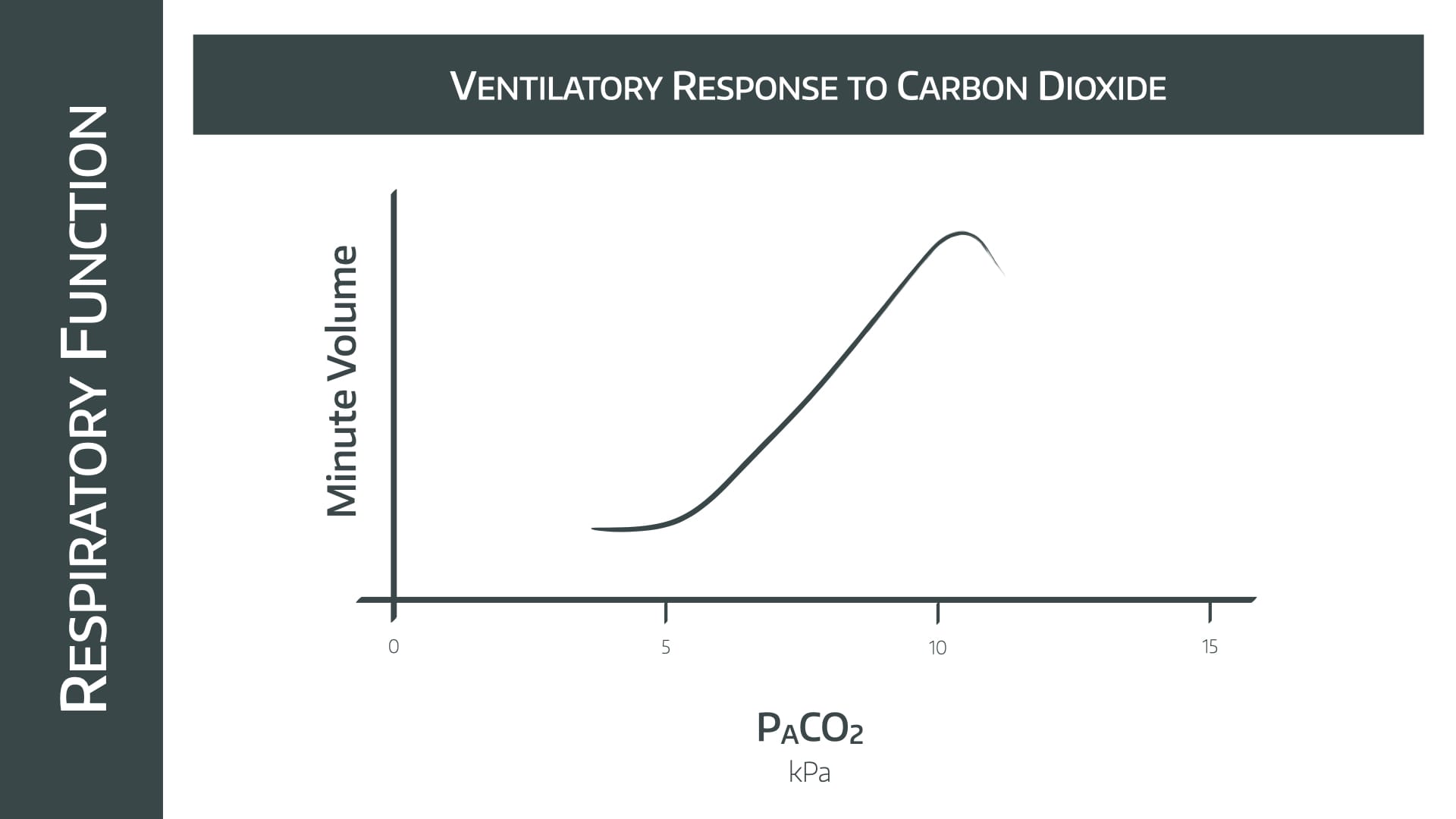
Note the relatively linear increase in minute ventilation as PACO2 increases between 5 and 10kPa
- Doubling the PACO2 causes the MV to increase by 4x
- Above 10kPa the effects of narcosis are seen
Changes that often get examined
- A raised threshold is seen in chronic CO2 retainers (COPD), which shifts the graph to the right
- Opioids cause reduced CO2 sensitivity, causing a right shift and vertical compression of the graph
A bit about the alveolar gas equation

There is one key point to take home from this - the level of CO2 in the blood will affect the amount of oxygen in the blood
This can be seen from the term on the right in the equation above - a higher PaCO2 will result in a lower PAO2.
Clinically this just means that if you want to optimise oxygen delivery to your patient's tissues, you don't want to let the PaCO2 climb too high.
Check out our full post on the alveolar gas equation here:
What happens if I stop breathing?
This is bad, clearly, but how bad?
- There are about 120 litres of CO2 in the blood in total, and the cells are churning out another 200 ml on top of that.
- This means your PaCO2 will rise by between 0.4 - 0.8 kPa each minute.
- This not only causes problems with pH, vasodilatation and narcosis but causes hypoxia via the alveolar equation.
Useful Tweets and Resources


References and Further Reading
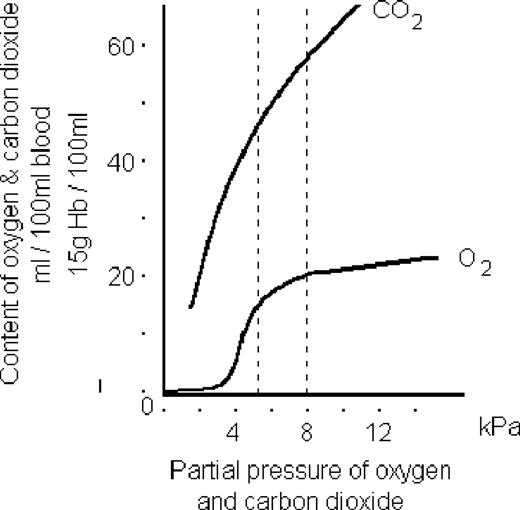
Primary FRCA Toolkit
Members receive 60% discount off the FRCA Primary Toolkit. If you have previously purchased a toolkit at full price, please email anaestheasier@gmail.com for a retrospective discount.
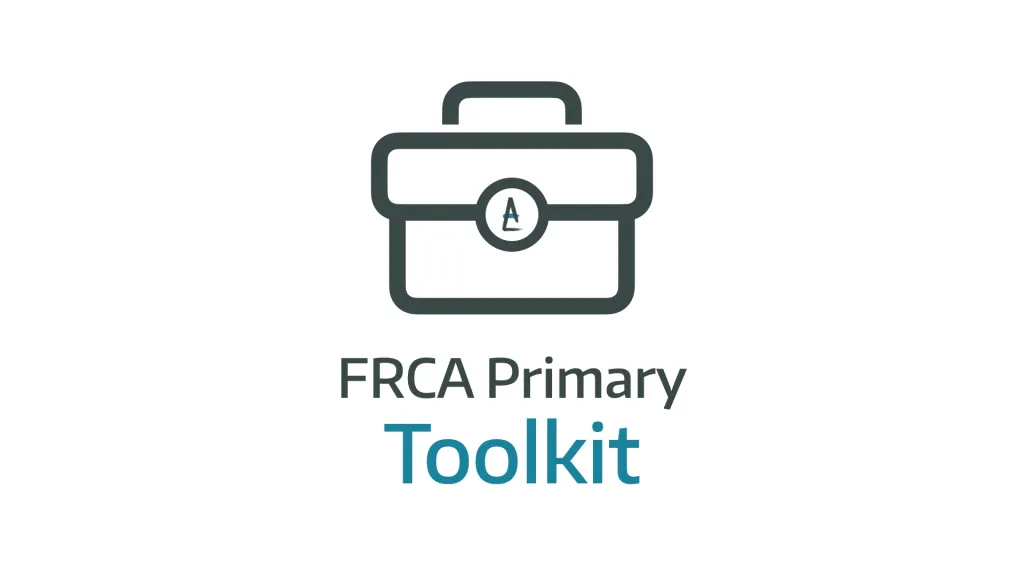
Discount is applied as 6 months free membership - please don't hesitate to email Anaestheasier@gmail.com if you have any questions!
Just a quick reminder that all information posted on Anaestheasier.com is for educational purposes only, and it does not constitute medical or clinical advice.